As is with any story, the story of neutrino oscillation will also be a journey. It will begin with the mystery behind sun's energy, encountering a myriad of questions and adversities, and ending at neutrino oscillations.
So let us begin!
The Mystery of Sun's Energy
The story starts during the 19th century when physicists were just discovering the fundamental laws of thermodynamics and energy conservation. The big question during the time was about the production of energy in the Sun. The initial theory was that meteors or asteroids falling into the Sun must be the reason for its energy! They theorized that the kinetic energy from the falling bodies was converted into heat energy.
Of course, the idea was a bust! Then there came the idea that it's probably the Sun’s small contractions that are producing the energy. The conversion in this case was from the gravitational energy (due to the contraction) into heat energy. It was called the Helmholtz- Thompson (Kelvin) contraction. This idea sounded more sane and to verify it, the age of the Sun was calculated corresponding to the energy. And guess what the number came out to be? 18 million years!
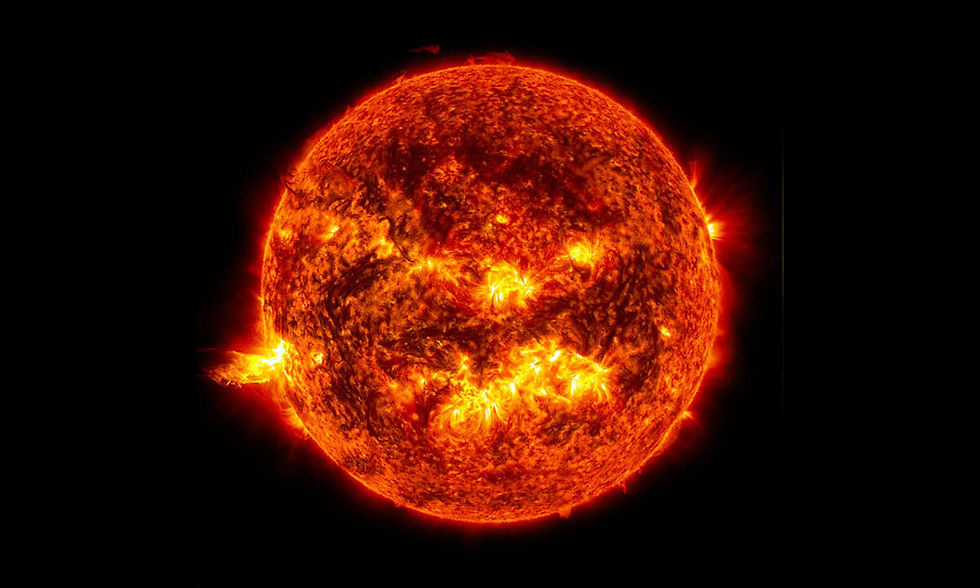
Now the problem was that in the 18th century, Charles Darwin (English naturalist and biologist) had estimated the age of the Earth to be 300 million years by careful geological evaluations. So obviously, the age of the Sun should not be just 18 million years!
It was in the 20th century when nuclear physics had made some progress that the correct idea became clear. In this case, the conversion of energy was from nuclear mass to the Sun’s energy. This is what the Solar Model was built upon. You can learn more about the Model itself here but the main idea behind the model was to account for various nuclear reactions in the Sun and the corresponding energy produced.
In the interiors of the Sun where the gravitational pressure provides immense energy, four Hydrogen nuclei can combine to form one Helium nucleus. Now, the process itself requires a large amount of energy to overcome repulsive forces between the Hydrogen atoms when they get too close to each other. So if the input energy itself is so large, then what is the reason behind the output energy being larger?

The answer lies in the fact that four Hydrogen nuclei (individually) are heavier than a Helium nucleus (M<m1+m2). This residual mass is what is responsible for the energy of the Sun (E=mc^2). There are many such nuclear fusion cycles taking place at different phases in a star’s life. It is in these nuclear reactions that a large number of neutrinos are produced. Neutrinos only interact via the weak force and hence pass through the Sun very quickly and reach us. At this very moment, we are bombarded with 100s of billions of solar neutrinos which aren’t even interacting with the matter in our bodies and just passing through.
The Homestake Experiment
In 1965, Raymond Davis Jr. (Ray Davis) (American Chemist and Physicist) began building an experiment to count the number of solar neutrinos. John Bahcall (American Physicist) gave the expected number of these solar neutrinos after a thorough theoretical analysis. The problem with neutrino detection is that they don’t interact with matter easily and only interact via the weak force. So the experiment had to take place in a large apparatus and away from any disturbances caused by other energetic particles.
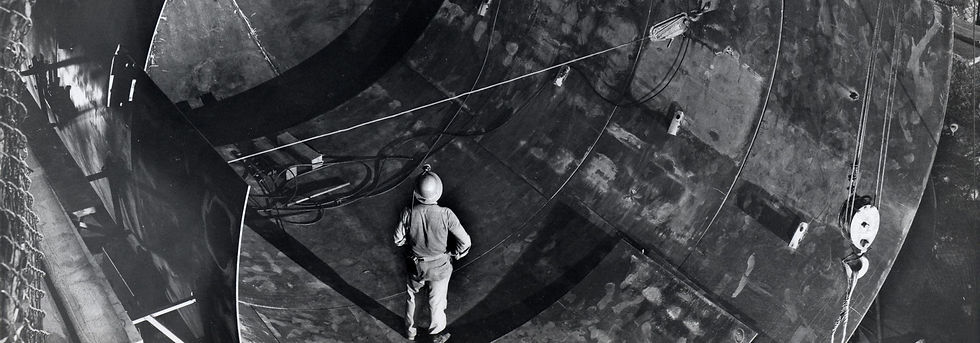
This experiment called the Homestake experiment, was built 4,850 feet (1,478 meters) underground in a 100,000-gallon tank of perchloroethylene (dry cleaning fluid). The solar neutrinos would interact with the Chlorine atoms and lead to Argon atoms as products. The number of Argon atoms in the liquid should therefore correspond to the number of solar neutrinos detected.

But when the result came, the number detected was between one-third to half of the expected value. The experiment and calculations were verified again but the results were the same. At this point, people weren’t sure if the problem was with the experiment, the Solar Model, or the theory of neutrinos itself. This discrepancy between the expected and observed number of neutrinos came to be called the Solar neutrino problem.
Pontecorvo's Solution
In 1957, Bruno Pontecorvo (Italian Nuclear Physicist) suggested something called neutrino oscillations. His theory didn’t receive much attention but when he heard the results of Ray Davis’ experiment years later, he pushed his theory again. Pontecorvo’s theory was based on his belief that as there were oscillations observed in the quarks due to weak force, so is possible in leptons as well.
So when antineutrinos were discovered, he could confidently put forth his theory of neutrino oscillations. Let us understand the early versions of neutrino oscillations better.
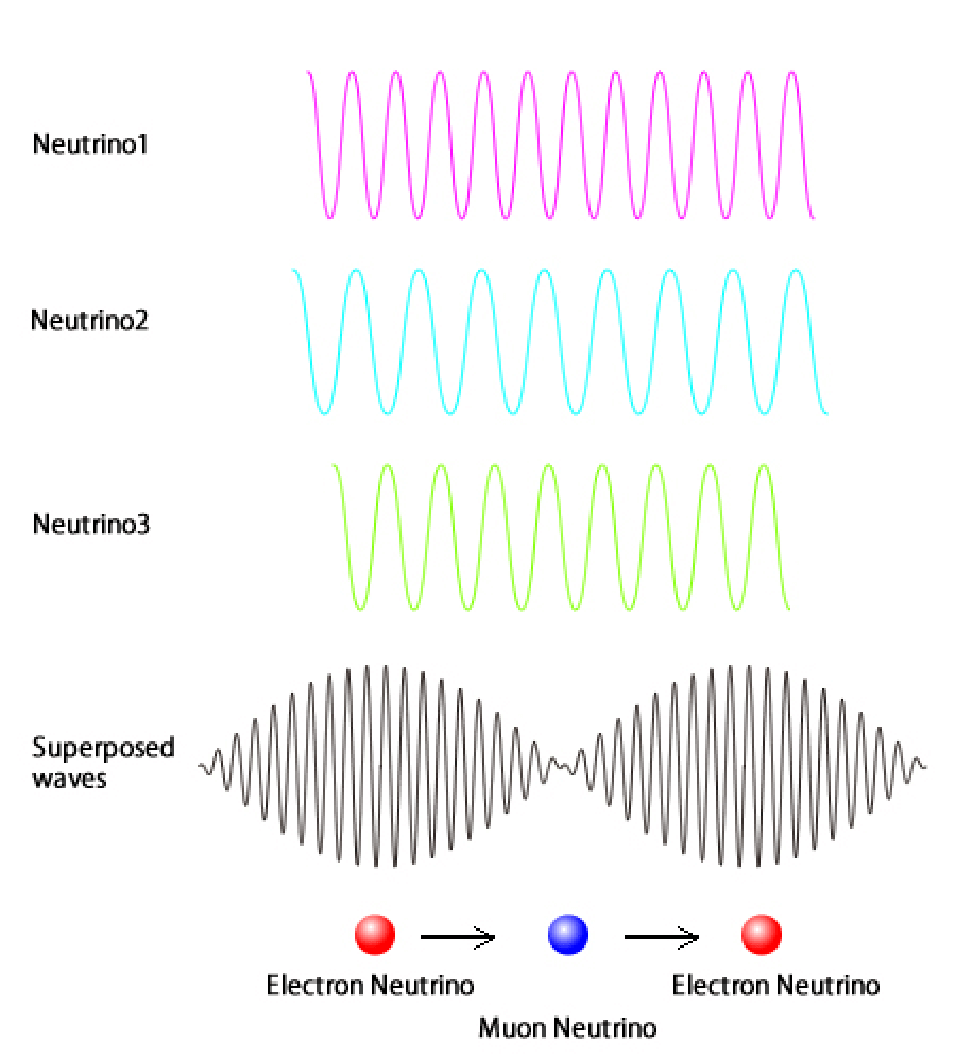
Remember that elementary particles show dual behaviours (wave and particle). Since neutrinos and antineutrinos are different particles, their waves had different frequencies when they travelled. Now each of these neutrinos and antineutrinos exist in superposition with each other. Meaning, that if a neutrino is produced in a reaction and is traveling in space, at a specific position in time, it will change to an antineutrino and then back into a neutrino, and so on. This will of course depend upon the superposition state of the wave at that point in time. This is what Pontecorvo explained as neutrino oscillations in 1957.
After the discrepancy in the Ray Davis experiment and further discovery of muon neutrinos (atmospheric neutrinos), Pontecorvo extended the neutrino oscillations to include muon neutrinos, meaning that neutrinos could not only change to their anti-neutrino states but also to different flavors.
But even though later experiments vindicated the Homestake experiment, the neutrino oscillations theory was still not accepted. Partly because for such a phenomenon to be true, neutrinos should have at least some mass. However, according to the Standard Model of particle physics, neutrinos didn’t have mass and travelled at the speed of light.
Atmospheric Neutrinos
When cosmic rays enter the Earth’s atmosphere, they interact with the molecules in the air and produce further particles. These particles are primarily pions. They are highly unstable and decay into muon and muon antineutrinos (or antimuon and muon neutrinos). These muon neutrinos/ antineutrinos make up about 2/3rd of the neutrinos in the atmosphere and hence are called atmospheric neutrinos.
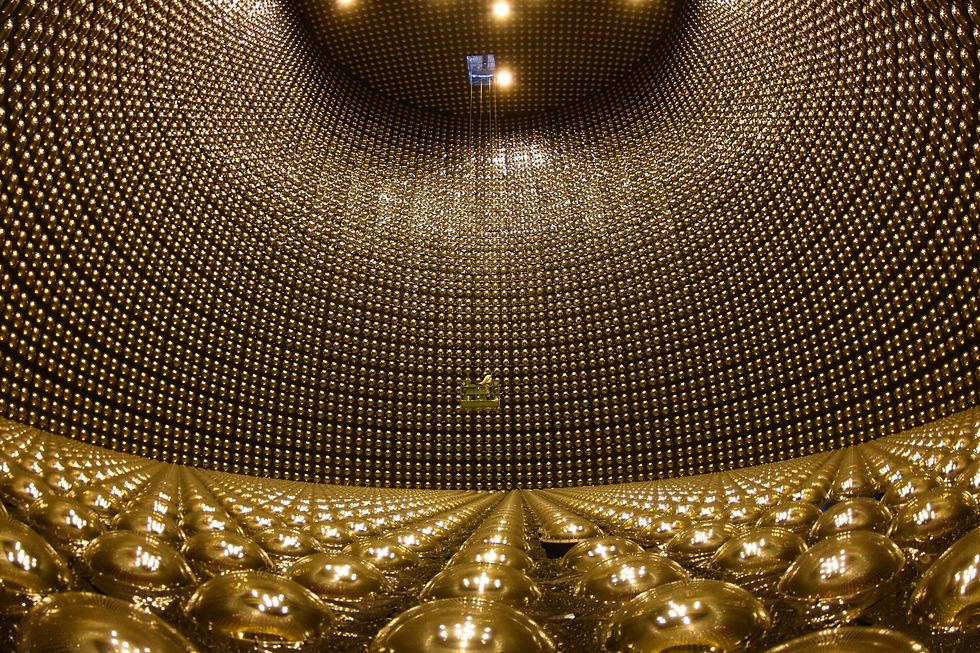
The Super Kamiokande detector was built in the 1990s to detect these muon neutrinos. Again, they had a certain number of neutrinos to be detected in mind. They detected the number of neutrinos coming from the other side of the Earth (crossing the Earth and reaching the detector) and those coming from above. But when the numbers rolled in, they found something strange!
The number of these muon neutrinos coming from across the Earth was only half that of coming from above. The only explanation was that the neutrinos were transforming into other types as they were traveling. This result was published in 1998 and created a lot of buzz because the peculiar behaviour observed had a name - neutrino oscillations.
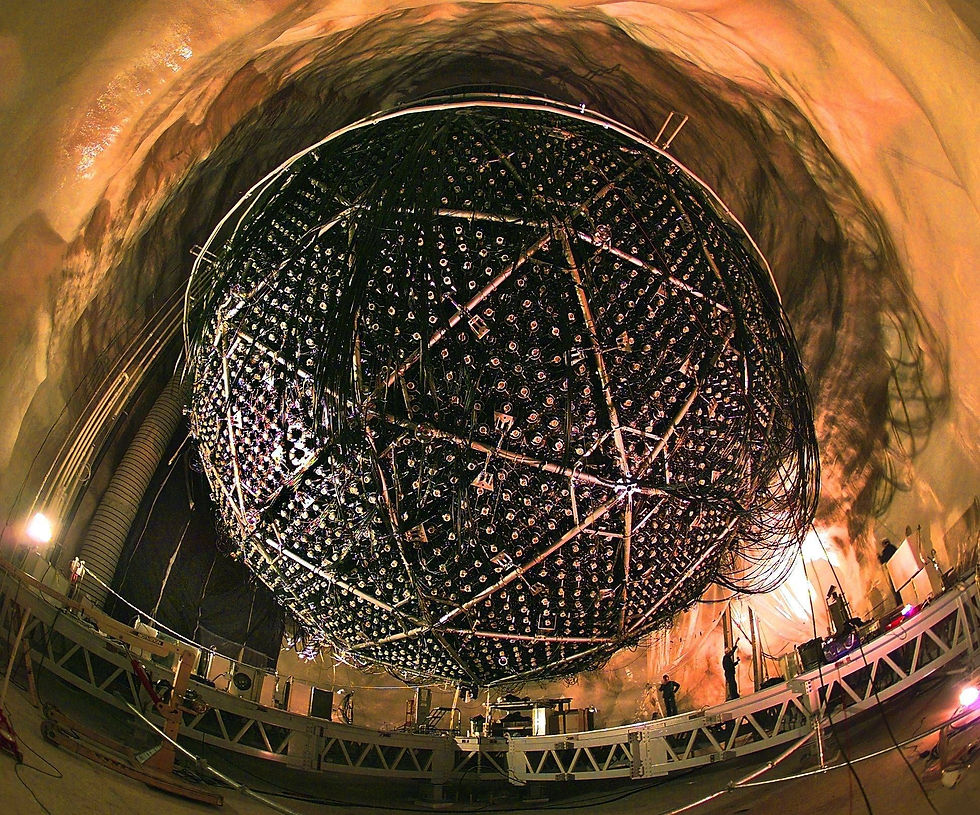
By 2001, even solar neutrino detection data from the Sudbury Neutrino Observatory (SNO) in Canada and Super Kamiokande was compared and it was official - Neutrino Oscillations were proved!
Neutrino Oscillations
There are three types of neutrinos - electron neutrino, muon neutrino, tau neutrino. Each is different from one another and produced in different reactions. But they all exist in a superposition state with each other. Let us understand it better with an example.
Imagine an electron neutrino being formed in the fission reactions of the Sun. As it propagates in space the phases of the wave will keep on changing. But since it's a superposition of three different waves (flavors of neutrinos), at a certain point the phase will be that of a muon or tau neutrino. This will keep on changing as the neutrino propagates. This behaviour is called the neutrino oscillation.
As is obvious, this implies that neutrinos aren’t massless as was proposed by the Standard Model theory, a theory which has up until then proven itself in countless discoveries of particles and their behaviours. This led to the realization that the Standard Model isn’t infallible after all and that there might be something more beyond it!
Glossary
Quarks: Elementary particles that are building blocks of more composite particles called hadrons, such as neutrons, protons. There are six types with each type having its own antiparticle. (Come under the category Fermion)
Leptons: Elecmentary particles with half integer spin (1/2). There are six types of leptons divided in three generations - electron, muon, tau and electron neutrino, muon neutrino, tau neutrino. Each of them have corresponding antiparticle.
Pions: Composite particles consisting of a quark and an anti-quark pair. There are three types of pions.
Comments